Synthetic DNA Advances Will Catalyze the Next Wave of Biotherapeutic Innovation
Recent innovations in DNA manufacturing are helping eliminate critical bottlenecks to bring new therapies to market faster than ever.
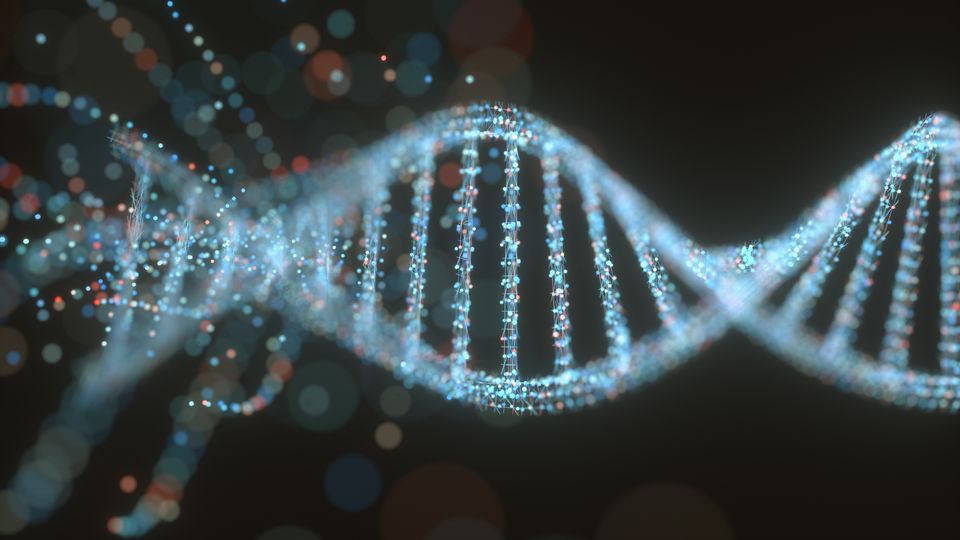
Complete the form below to unlock access to ALL audio articles.
With ever-increasing competition, biopharmaceutical companies face significant challenges in swiftly bringing new therapeutics to market while effectively managing their capital and resources. In 2022, top biopharmaceutical companies allocated an average of over $2 billion to launch a single therapy from R&D through clinical trials to the market, highlighting the immense investment involved.1 2022 also saw FDA approvals for biopharmaceuticals pushed ahead of small molecules for the first time.2 In comparison to traditional small-molecule drug production, biopharmaceuticals are more sophisticated and difficult to scale, as their manufacturing processes necessitate specialized facilities operated by highly skilled personnel, with annual operational expenses reaching between $37.3 to $55.5 million annually.3 For some advanced therapeutic medicines, the cost to manufacture each therapy can be as high as $500,000.4
To maintain their leadership position and ensure a favorable return on investment, industry leaders must continually reassess their operational strategies and embrace innovative technologies to streamline processes, eliminate bottlenecks, reduce variability and enhance the speed and reliability of their development workflows. One ubiquitous and indispensable biomanufacturing input ripe for improvement is a scalable, turnkey solution for high-quality synthetic DNA. DNA serves as the programmatic code for every living cell and plays a crucial role in the development of all biopharmaceuticals, including mRNA vaccines, cell/gene therapies and precision medicines.
A fast and reliable supply of DNA is imperative for biopharmaceutical companies to ensure the overall efficiency and success of their operations. However, manufacturing DNA that meets the necessary length, accuracy, complexity, purity and scale requirements to advance therapeutic candidates from discovery to the market has proven to be anything but turnkey.
Recent innovations in DNA manufacturing are helping eliminate critical bottlenecks to bring new therapies to market faster than ever.
The cell-based DNA synthesis bottleneck
In the early stages of development, biopharmaceutical candidates heavily depend on an iterative design-build-test-learn (DBTL) cycle. This cycle involves testing and re-designing synthetic DNA constructs until potential therapeutic candidates are identified. However, conventional manufacturing methods often result in lengthy, weeks-long production times, especially for longer and more complex DNA constructs. Consequently, researchers spend a significant amount of time building DNA or waiting for suppliers to do so, delaying the crucial task of testing hypotheses and optimizing candidate therapies.
A closer look at mRNA therapy
A clear example of the DNA supply bottleneck can be found in the development of mRNA therapeutics produced by in vitro transcription (IVT), a process whereby a linearized DNA sequence template is transcribed into mRNA. The conventional production of long, double-stranded DNA templates starts by assembling short, single-stranded oligonucleotides of 60–100 bases into short, double-stranded DNA fragments, typically up to 2,000 base pairs (bp) long.5 Due to errors inherent in the synthesis methods used, the short fragments produced are not pure, often containing molecules with errors in the DNA sequence. Manufacturers use a weeks-long process to correct the errors in the fragments and assemble them into a cloning vector, which is then propagated in bacterial cells that are ultimately plated on agar plates to grow into colonies. Bacterial colonies are sampled and sequenced to identify a sequence-perfect clone. Once a colony carrying a sequence-perfect plasmid is selected, the plasmid DNA is isolated from the cells, linearized and purified to serve as a template for mRNA synthesis by IVT.
The task of linearizing and purifying plasmid DNA prior to IVT should not be regarded as trivial by any means. Inefficient linearization of the plasmid can result in the expression of backbone DNA containing antibiotic-resistant markers. This, along with any remnants of host DNA or other contaminants from cell-based cloning, must be removed prior to IVT, adding time and cost to the process.
The entire process is then repeated to test each new DNA template design, taking months to identify candidates for preclinical studies. For therapeutics using self-amplifying RNA and trans-amplifying RNA formats, the frequency of design iteration can be higher than traditional mRNA designs, taking even more time. Speed is critical in the biopharmaceutical industry, and waiting weeks for DNA for each iterative development round is not an optimal solution.
The challenge of scaling mRNA vaccine production
After a therapeutic candidate has been identified and the decision is made to move into clinical testing, large quantities of plasmid DNA, manufactured under GMP compliance, are required to synthesize enough mRNA to perform clinical studies and treat patients. For this, conventional GMP workflows rely on the same weeks-long, tedious and cumbersome molecular cloning process, as well as two to three months of initial studies to select, characterize and establish a master cell bank (MCB), a large population of cells that serve as a seed stock. GMP DNA is then isolated, purified, linearized and transcribed from the MCB into large quantities of mRNA using IVT.
While cell-based processes are arguably cost-effective for high-scale amplification, they require months for processing and are plagued with many problems, batch-to-batch variability, extensive purification and testing, and potential downstream toxicity resulting from residual cell debris post-cellular lysing. Not to mention that many desired therapeutic constructs are toxic to bacterial hosts, preventing them from being manufactured in the first place, despite their potentially superior therapeutic performance. Realizing that they cannot continue to rely on antiquated processes, forward-thinking organizations are exploring DNA manufacturing innovations that eliminate conventional cloning, large fermentation tanks and the rising costs of associated infrastructure and labor.
New innovations enable cell-free DNA synthesis
Surprisingly, there has been relatively little innovation in conventional DNA synthesis over the past several decades. Recent years have seen some incremental innovations that address singular steps of the build workflow. However, although promising, these singular improvements fail to deliver a meaningful impact on the performance of the overall DNA manufacturing process.
Synthetic DNA is produced by a continuous build process to transform single, low-quality oligonucleotides and fragments into longer DNA and genes. Therefore, innovating a single step, such as oligonucleotide synthesis, only to then use conventional cloning to build full-length DNA or scale the production of DNA is not an effective solution. No singular innovation can solve the DNA supply bottleneck in genetic medicine development. Radical changes are needed throughout the DNA production process, not least of which includes the cell-based cloning step, used by synthetic DNA manufacturers for the past several decades.
Recently, new suppliers have emerged with a focus on producing long, double-stranded DNA in novel ways. These companies use innovative cell-free synthesis, assembly and cloning strategies to accelerate and scale full-length DNA production. The result is sequence-perfect, multi-kilobase, double-stranded linear DNA that can be manufactured in days and used in therapeutic workflows with minimal or no additional cloning. Companies have received NGS-verified, high-complexity DNA as long as 7,000 bp in less than a week, ready for immediate use downstream without further processing. With this length and accuracy, even long gene constructs, e.g., 20,000 bp, can be assembled within two weeks. With accuracy nearly equivalent to plasmid DNA, the high-quality linear DNA can be used directly in IVT workflows, eliminating the weeks of time needed to clone, purify and linearize plasmid DNA.
With an agnostic approach to oligonucleotide synthesis chemistry and cell-free cloning technology, this groundbreaking innovation in DNA manufacturing offers unparalleled scalability and cost-efficiency compared to traditional methods. It is revolutionizing the discovery and development of mRNA therapies and will enable rapid scale-up of therapeutic candidates for preclinical and clinical studies. By eliminating bottlenecks in genetic medicine workflows, this technology not only accelerates the development process but also ensures the delivery of full-length, high-quality DNA free from cellular contaminants. Looking beyond mRNA biotherapies, this capability is particularly crucial for individualized therapy, where the timely production of safe and effective treatments, devoid of cellular contaminants, can significantly extend patients' lives.
Conclusion
The COVID-19 pandemic and the development of its mRNA-based vaccines brought attention to the need for faster healthcare innovations to meet medical challenges. Indeed, companies are racing to apply this technology to prevent and treat many illnesses, including influenza, shingles, HIV and cancer. Biopharmaceutical organizations seeking to remain competitive must adopt innovations, like cell-free long DNA synthesis, to unleash the potential of synthetic biology and accelerate therapeutic development across all facets of genetic medicine.
About the author:
Dan LaCaze is chief business officer at Elegen.