Synthetic Biology: Nature's Tools, Redesigned
Can synthetic biology help scientists tackle global issues such as climate change and undruggable diseases?
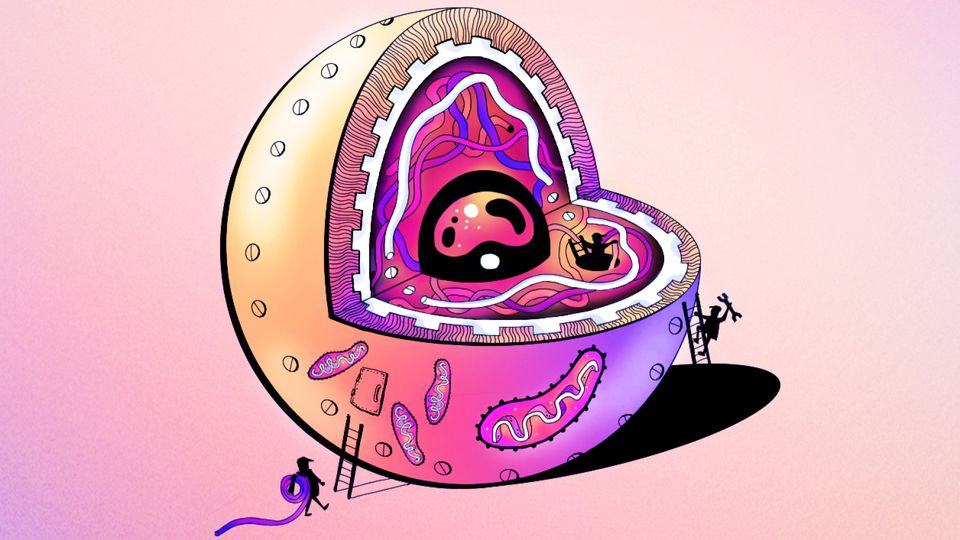
Complete the form below to unlock access to ALL audio articles.
Synthetic biology is a multidisciplinary field that remodels organisms for useful purposes by engineering them to have novel abilities, often drawing inspiration from nature to build molecular components that don’t exist in the natural world.
It uses living organisms to solve problems by breaking them down into individual components and applies engineering principles to coordinate changes to RNA, DNA and proteins to form biological systems with novel functions. These systems can be designed based on known biology, or from scratch, to carry out a certain task.
“I like a simple definition, that contrasts analytic biology, the study of living systems that already exist in nature, with synthetic biology, which makes new or modified living systems that do not exist in nature,” says Dr. Jamie A Davies, professor of experimental anatomy at the University of Edinburgh. “This links nicely with the dichotomy between analytical chemistry, the study of what already is, and synthetic chemistry, which makes something new.”
Synthetic biology has several useful applications, in conservation for example, to restore biodiversity and confer disease and stress resistance, in bioremediation to clean pollutants from the air and water, in manufacturing, agriculture and farming and in biofuels.
In medicine, synthetic biology is helping scientists to better understand diseases and treatment. It can be used to produce drugs and new vaccines, improve monitoring and diagnosis and alter human cells with properties designed to help patients. Research is also turning its attention to building new tissues.
Synthetic biology and therapeutics
Nature is an important source of bioactive products, and therefore often a source of new therapeutics; over the last 30 years most anticancer, anti-infective and anti-bacterial drugs originated from natural sources, like plants, fungi or bacteria, and their derivatives. But developing a new therapeutic is time-consuming, expensive and challenging; it can take 10 to 15 years from target identification to approval, and cost as much as $2.9 billion per drug, with only 1 in 5,000 targets making it to market.
Synthetic biology can aid the design of new therapeutics in two broad ways, says Davies: “One is epitomized by CAR T cells used to treat cancer: engineering human cells to have new properties that are useful, for example, the ability to mount a ferocious attack on a patient's tumor, and put them back into the body to do that job.”
CAR T, or Chimeric Antigen Receptors T-Cell Therapy, is a highly complex and innovative type of immunotherapy that harnesses a patient’s own immune system to target cancer cells. T cells or lymphocytes are harvested from the patient’s blood and genetically engineered in the lab to recognize and bind to specific proteins, or antigens, on the surface of cancer cells, before eradicating them.
These therapies initially focused on acute lymphoblastic leukemia (ALL), the most common cancer in children, but have the ability to eradicate very advanced leukemias and lymphomas, and can keep cancer at bay for several years after infusion.
The second way that synthetic biology can aid drug discovery, says Davies, is engineering cells to perform the physiological role of another, for example, non-pancreatic cells to make insulin in response to blood sugar. This application is currently experimental and not yet used in humans.
“People with type 1 diabetes destroy natural pancreatic cells because of an autoimmune problem, but do not destroy other cells, like skin cells, so engineering these other cells to do the insulin job would cure diabetes,” he says. Scientists at the J. Craig Venter Institute have engineered bacterial cells that naturally live deep within the skin to function like insulin cells, which entered preclinical testing in 2021. “And you can extend this idea to many similar diseases,” Davies says.
The other broad way is using engineered metabolic pathways to produce difficult-to-make drugs, notes Davies: “The real example in current use is engineering production of the antimalarial drug artemisinin into yeast cells, by 'borrowing' genes from plants to build up a new metabolic pathway in the yeast. And this idea could be extended to all sorts of other molecules.”
The main source of artemisinin is extracted from Artemisia annua; however, its artemisinin content is only 0.1–1% – nowhere near enough to meet global demand. The chemical synthesis of artemisinin is complicated, expensive and offers a low yield, so the ability to produce artemisinin in vivo using synthetic biology is attractive.
Researchers have explored the option of synthesizing artemisinin using Escherichia coli (E. coli) and Saccharomyces cerevisiae (S. cerevisiae) as chassis cells – a cellular host or recipient of engineered biological systems in synthetic biology – and yeast as the cell factory, thus transferring the production of artemisinin from plant to yeast.
Synthetic biology is highly and rapidly adaptable, making it ideal to respond to urgent needs like vaccines. An approach focusing on large-scale nucleic acid manipulation was successfully used to create a COVID-19 vaccine, while genomic codon deoptimization is used to create injectable and nasal vaccines against the flu and respiratory syncytial virus. Other vaccines are based on introducing DNA and RNA components encoding viruses into human cells. These cells produce viral antigenic peptides in a repetition of the natural infectious process to induce immunity.
Synthetic biology and diagnostics
Synthetic biology also has a role to play in diagnosing disease; systems can be introduced into cells to sense biological signals and chemical substances within the cell, and even respond to stimuli.
Davies says synthetic biology’s role here is to engineer cells or proteins to be sensitive and accurate sensors of markers of disease: “This might include a simple test, a drop of blood or urine, or even a patch on the skin, to report all the time to a reader that can talk to a mobile phone and let doctors monitor how someone is doing. These sensors offer the promise to be combinatorial too – to measure many things at once.”
Techniques based on gene circuit construction and rapid, iterative prototyping have enabled the development of devices, ranging from whole-cell living assays to engineered cell-free nucleic acid sensors and amalgams in between, which have been applied to non-communicable diseases such as cancer and coronary artery disease, and communicable diseases.
One area of active research is paper-based toehold switch RNA sensors. RNA toehold switch sensors are robust molecular switches that can detect RNA molecules in a sequence-specific way, and when combined with a paper-based cell-free molecular diagnostic platform, are capable of detecting Ebola, Zika virus, COVID-19 and other respiratory diseases.
Another is the use of CRISPR-Cas technology as a rapid molecular diagnostics tool for nucleic acids and detection of pathogens. The technique enables researchers to target specific stretches of genetic code and to edit DNA at precise locations,.
Each disease-causing virus or microbial pathogen has a characteristic sequence of nucleic acids. CRISPR-Cas technology can recognize and snip this specific nucleic acid sequence in a sample, meaning it can be used as a diagnostic biomarker. Screening based on CRISPR-Cas can be helpful in searching for biomarkers that identify populations sensitive to a certain cancer treatments, for example.
Synthetic biology and agriculture
CRISPR isn’t just limited to medical applications; it also has a role to play in farming and agriculture, where it is an effective technique for manipulating a number of crops, including maize, wheat and apples.
Genetic editing can be used to introduce desirable qualities into plants in a faster, controlled and specific way. It allows scientists to make very precise changes to the plant’s DNA to confer traits, such as immunity or resistance against a certain pests or pathogens, to change how the plant grows or to change the soil microbiome to help improve crop yield and quality.
“Synthetic biology approaches, like genetic editing, can help improve food security, increase the yield and the resilience of crops in the face of climate change,” says Professor Alistair McCormick, chair of plant engineering biology at the University of Edinburgh. “The population is expected to reach 10 billion by 2050, and we need to improve all of these to feed the growing population.”
Genetic editing gives us the ability to grow the same crops on the same amount of land, but with a better yield, says McCormick, citing the recent example of knocking out the gene KRN2 in rice and maize, which led to 8 and 10% increases in grain yields, using thanks to gene editing.
The technique also allows scientists to alter the characteristics of staple crops like rice, which have been modified to produce beta carotene, a nutrient typically associated with preventing vitamin A deficiency , and to develop a type of wheat less likely to produce acrylamide, a potentially carcinogenic compound, during cooking or baking. This wheat has recently been subject to its first ever field trial and showed a significant reduction of acrylamide when the flour is baked.
Synthetic biology can also be used to produce healthier or specifically designed food ingredients, like high-value proteins, lipids and vitamins. Food production by microbes is considered a promising alternative that could allow food to be produced quickly in an environmentally friendly way.
However, such techniques will not be enough to meet the growing demand for food, McCormick adds – increasing crop productivity is essential for global food security. His work focuses on photosynthesis and how it can be manipulated to produce novel products or improve plant productivity. McCormick’s group aims to improve photosynthetic CO2 assimilation by engineering algae to increase photoassimilation rates and therefore productivity.
He notes that growing algae and heterotrophic species together is a “hot new research topic”. If these species can be grown together, then CO2 could be captured, converted into sugar and then used by the cell media. Researchers at the University of Michigan recently created a strain of cyanobacteria that can export up to 85% of the carbon it fixes as sucrose. Their approach, they say, “is promising as a potential alternative to land-based crops for sugar production, as this level of productivity could exceed sugar output from sugarcane or corn if it could be scaled up.”
Another “hot topic” is that of engineered probiotics designed to improve health by increasing the specificity of probiotic microbes or improving the antimicrobial activity of the probiotic against pathogenic infections of the gastrointestinal tract. Synthetic biology approaches have been used to engineer the probiotic bacteria, Lactobacillus johnsonii (L. johnsonii) to be effective against Clostridium perfringens (C. perfringens) a bacteria that causes food poisoning.
Synthetic biology’s impact, now and in the future
Davies says synthetic biology's greatest current strength is that it allows scientists to test their understanding: “In the same way that you can test your understanding of aerodynamics by making simple paper planes, we can test our understanding of complex biology by making simple things that work according to the principles we have derived, and we can see if they really work.”
“And when they don't, that tells us we have not really understood things at all and need to go back and do better analytic science,” he adds. Davies believes tools like CAR T will continue to grow and will have greater impact, as will non-medical applications, like organisms engineered to clean up water, which are important for health. “But most of this is still very expensive and experimental, and I think it will be a while before we see real impacts,” he says. “For now, the impacts are in research itself.”
McCormick believes synthetic biology will evolve into engineering biology – a move already being championed by UKRI. He says it will become more inclusive of branches like bioengineering and increase its remit to chemistry, physics and material science. The biggest impact, in his opinion, will be “in high value products, in pharma and in vaccines”.
“And it will make a big difference in agriculture to ensure we get as much as we can out of what we have. It may not solve the problem, but it will certainly help ease it,” he concludes.